Lena Noack
University professor, Freie Universität Berlin
Main research interests
- Simulation of mantle convection, volcanic activity, crust formation and plate tectonics
- Influence of mineral composition, thermodynamic properties and redox state on global planetary processes
- Coupling between interior, surface and atmosphere for terrestrial planets
Research profile at FU Berlin
Positions
-
Since 05/2021: University (W2) professor
Freie Universität Berlin, Department of Earth Sciences, Berlin, Germany
-
06/2017-04/2021: Junior professor
Freie Universität Berlin, Department of Earth Sciences, Berlin, Germany
-
10/2012-05/2017: Post-doc position
Royal Observatory of Belgium, Department Reference systems and Geodynamics, Brussels, Belgium
-
10/2008-09/2012: Doctoral studies
German Aerospace Center (DLR), Institute for Planetary Research, Berlin, Germany
|
|
Freie Universität Berlin
Department of Earth Sciences
Malteserstr. 74-100
Room D210 - Building D
12249 Berlin
Tel.: +49 (0) 30 838 636 94
Fax: +49 (0) 30 838 4 636 94
E-mail: lena.noack (at) fu-berlin.de
|
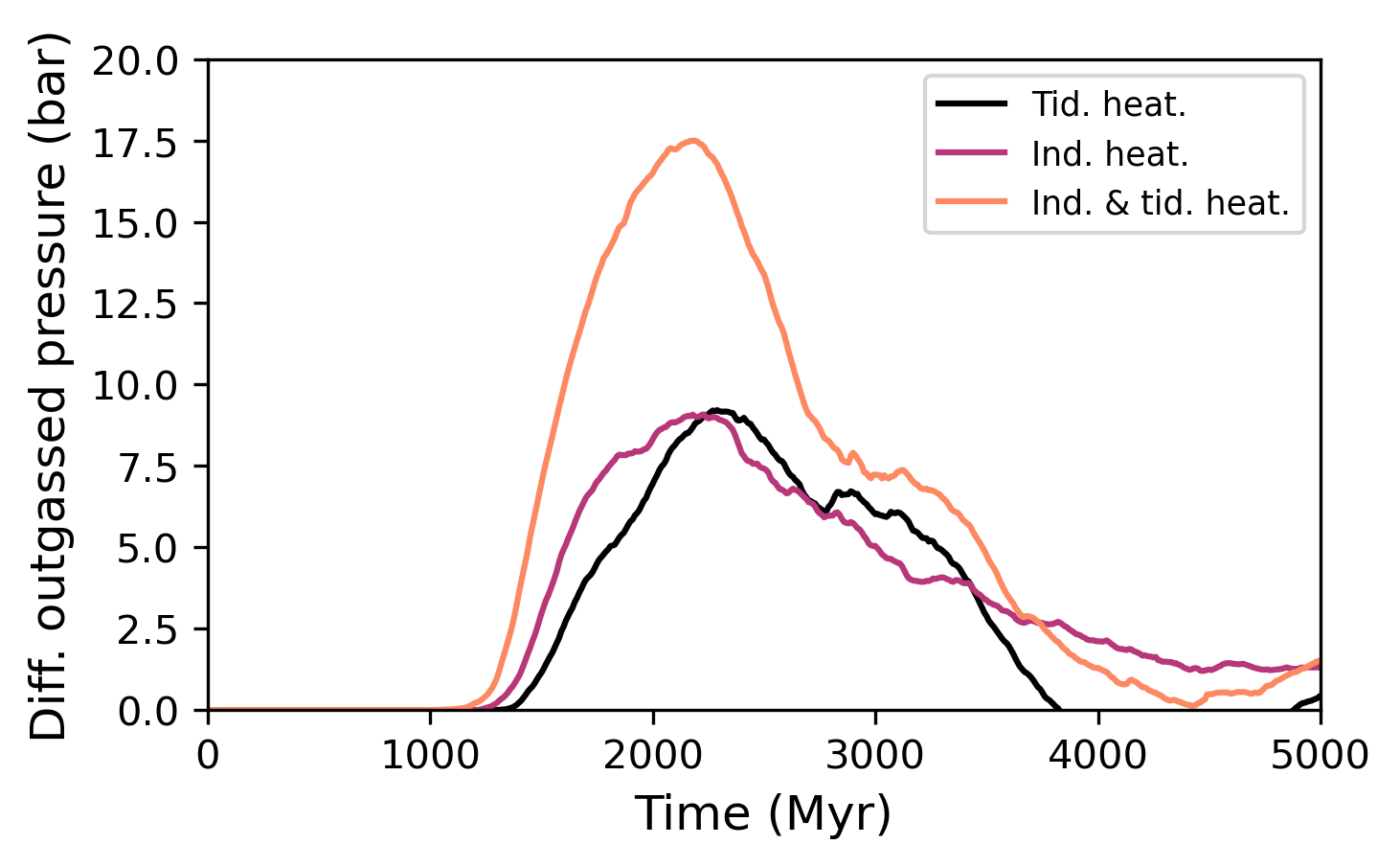 |
Interior heating and outgassing of Proxima Centauri b
Since the discovery of a potentially low-mass exoplanet around our nearest neighbour star Proxima Centauri, several works have investigated the likelihood of a shielding atmosphere and therefore the potential surface habitability of Proxima Cen b. However,
outgassing processes are influenced by several different (unknown) factors such as the actual planet mass, mantle and core composition, and different heating mechanisms in the interior.
We consider heating in the interior due to time-dependent radioactive heat sources, induction heating from the evolving stellar magnetic field, as well as tidal heating. We show that induction heating may have been significant in the past, leading to local temperature increases of several hundreds of Kelvin. This early heating leads to an earlier depletion of the interior and volatile outgassing compared to if the planet had not been subject to induction heating. We show that induction heating has an impact comparable to tidal heating when assuming latest estimates on its eccentricity. Furthermore, we find that the planet mass (linked to the planetary orbital inclination) has a first-order influence on the efficiency of outgassing from the interior.
|
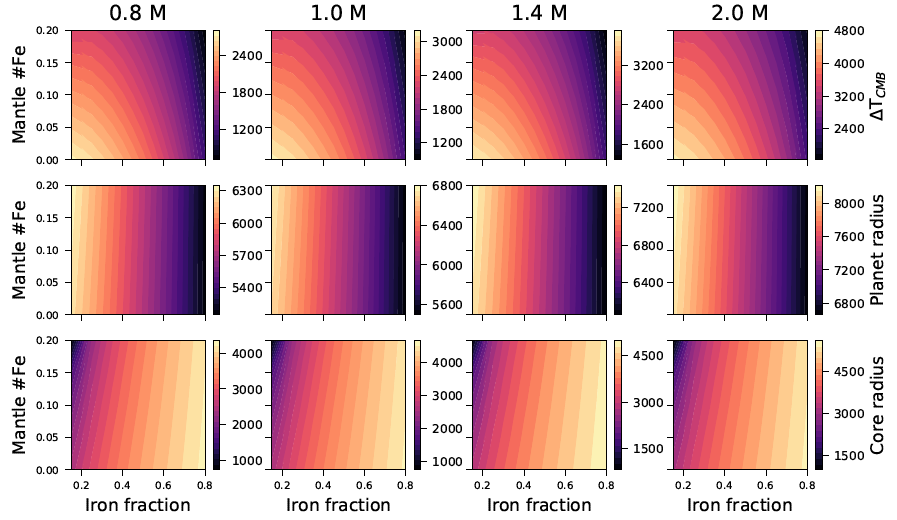 |
Parameterisations of interior properties of rocky planets
The observations of Earth-size exoplanets are mostly limited to information on their masses and radii. In this work, we investigate the structure of a rocky planet shortly after formation and later stages of thermal evolution assuming the planet is differentiated into a metal core and a rocky mantle (consisting of Earth-like minerals but variable iron number). We derive possible initial temperature profiles after the accretion and magma ocean solidification. We then develop parameterisations for the thermodynamic properties inside the core depending on planet mass, composition and thermal state. We provide the community with robust scaling laws for the interior structure, temperature profiles and core- and mantle-averaged thermodynamic properties for planets composed of Earth’s main minerals but with variable compositions of iron and silicates.
The scaling laws allow to investigate variations in thermodynamic properties for different interior thermal states in a multitude of application such as deriving mass-radius scaling laws or estimating magnetic field evolution and core crystallisation for rocky exoplanets.
|
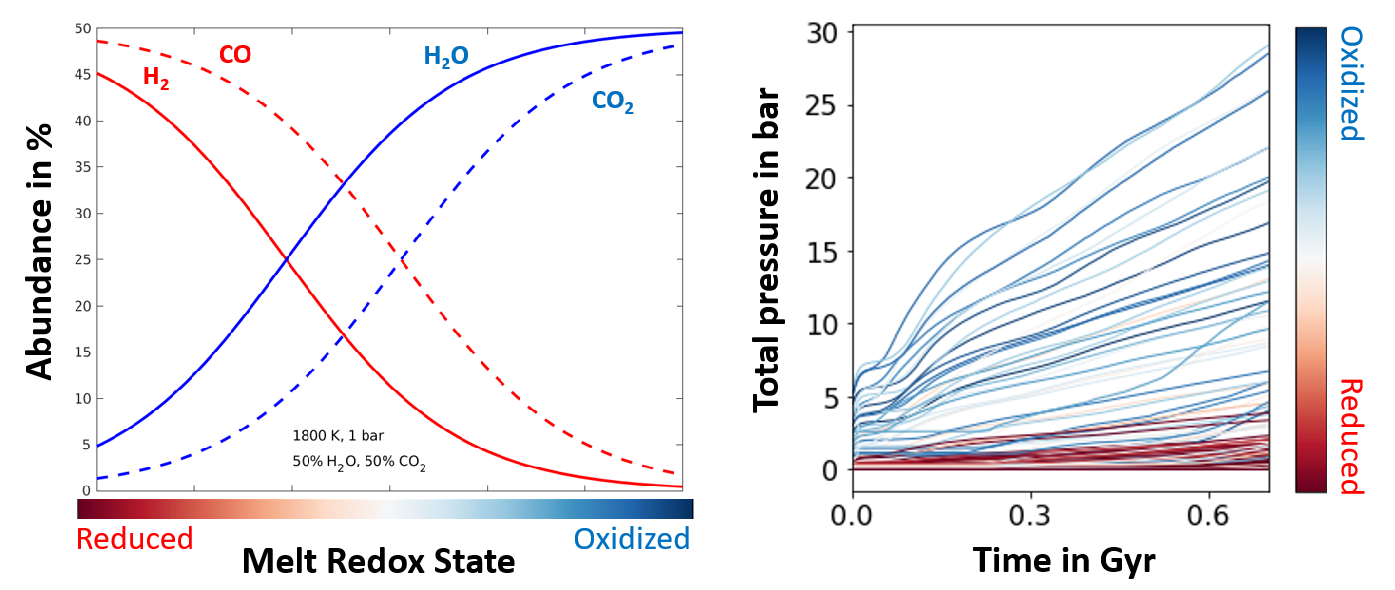 |
The redox state of the mantle is the main factor influencing volcanic outgassing
We coupled a numerical mantle convection model, a petrologic melting model, and a gas speciation model to simulate the
evolution of CO2, CO, H2O, and H2 outgassing for rocky planets. We find that the oxygen fugacity
of the mantle is by far the most important control on the outgassed mass and abundance of these species, followed by
the mantle thermal state and the initial mantle water content. The speciation between H2 and H2O shows a gradient in redox, while the speciation between CO and CO2 shows a gradient in partitioning during melting.
|
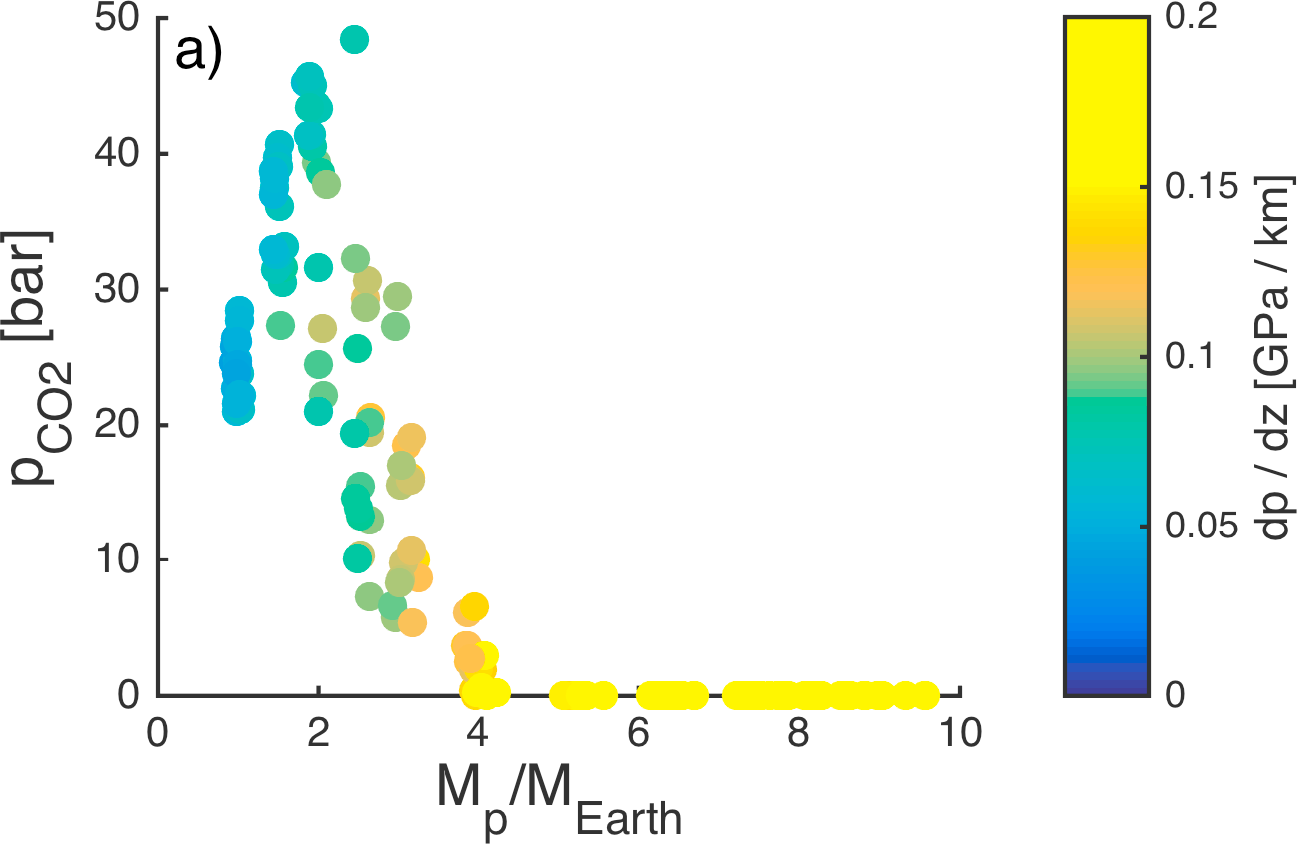 |
Outgassing on stagnant-lid super-Earths
We explore volcanic CO2-outgassing on purely rocky, stagnant-lid exoplanets of different interior structures, compositions, thermal states, and age. We focus on planets in the mass range of 1-8 Earth masses. We derive scaling laws to quantify first- and second-order influences of these parameters on volcanic outgassing after 4.5 Gyr of evolution.
Given commonly observed astrophysical data of super-Earths, we identify a range of possible interior structures and compositions by employing Bayesian inference modeling.
In total, we model depletion and outgassing for an extensive set of more than 2300 different super-Earth cases. We find that there is a mass range for which outgassing is most efficient (2-3 Earth masses, depending on thermal state) and an upper mass where outgassing becomes very inefficient (5-7 Earth masses, depending on thermal state). In summary, depletion and outgassing are mainly influenced by planet mass and thermal state. Interior structure and composition only moderately affect outgassing rates. The majority of outgassing occurs before 4.5 Gyr, especially for planets below 3 Earth masses.
These findings and our provided scaling laws are an important step in order to provide interpretative means for upcoming missions such as JWST and E-ELT, that aim at characterizing exoplanet atmospheres.
|
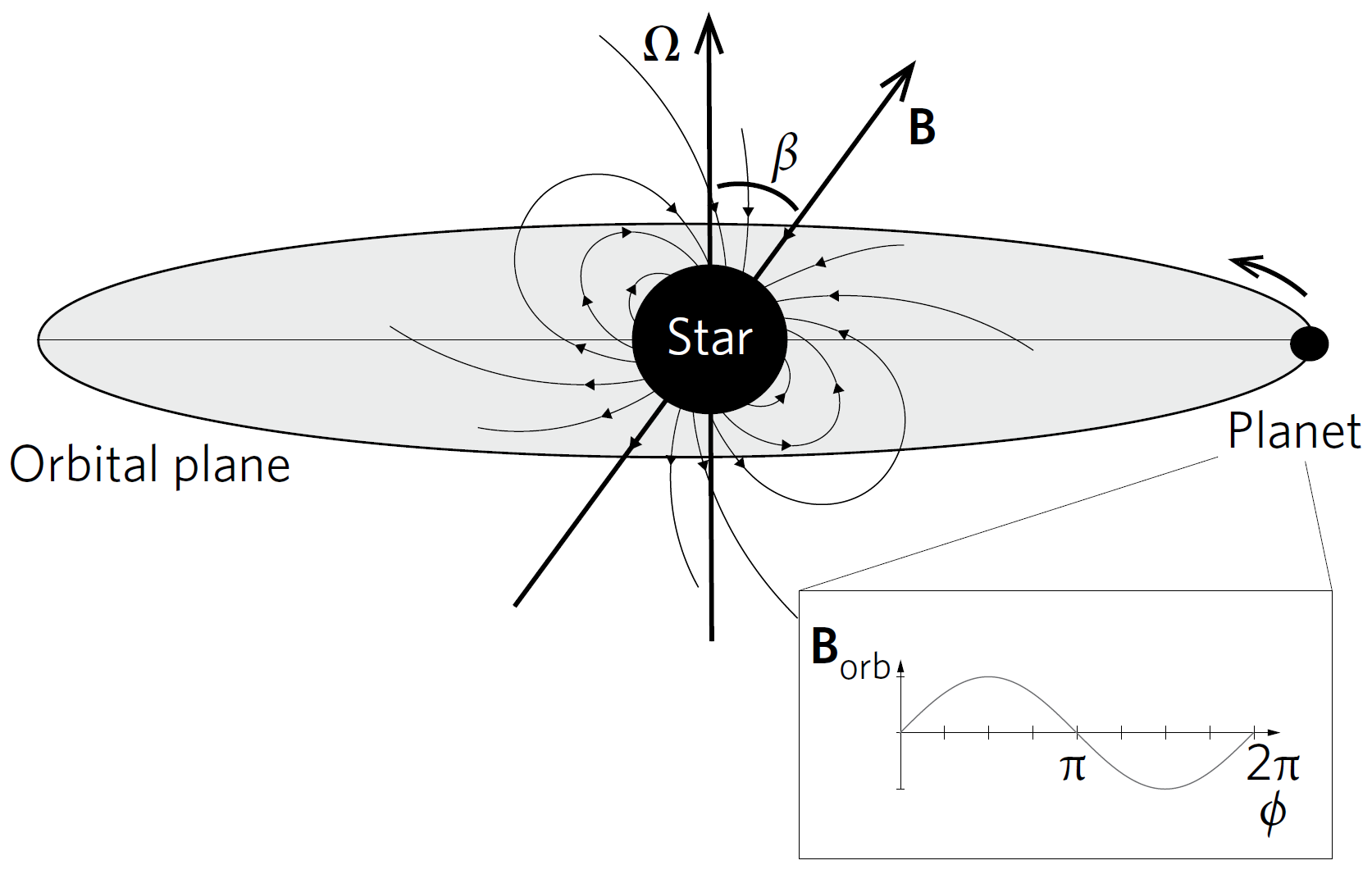 |
Magma oceans and enhanced volcanism on TRAPPIST-1 planets due to induction heating
Low-mass M stars are plentiful in the Universe and often host small, rocky planets detectable with current instrumentation. These stars host magnetic fields, some of which have been observed to exceed a few hundred gauss. Recently, seven small planets have been discovered orbiting the ultra-cool M dwarf TRAPPIST-1, which has an observed magnetic field of 600 G. We suggest electromagnetic induction heating as an energy source inside these planets. If the stellar rotation and magnetic dipole axes are inclined with respect to each other, induction heating can melt the upper mantle and enormously increase volcanic activity, sometimes producing a magma ocean below the planetary surface. We show that induction heating leads the four innermost TRAPPIST-1 planets, one of which is in the habitable zone, either to evolve towards a molten mantle planet, or to experience increased outgassing and volcanic activity, while the three outermost planets remain mostly unaffected.
|
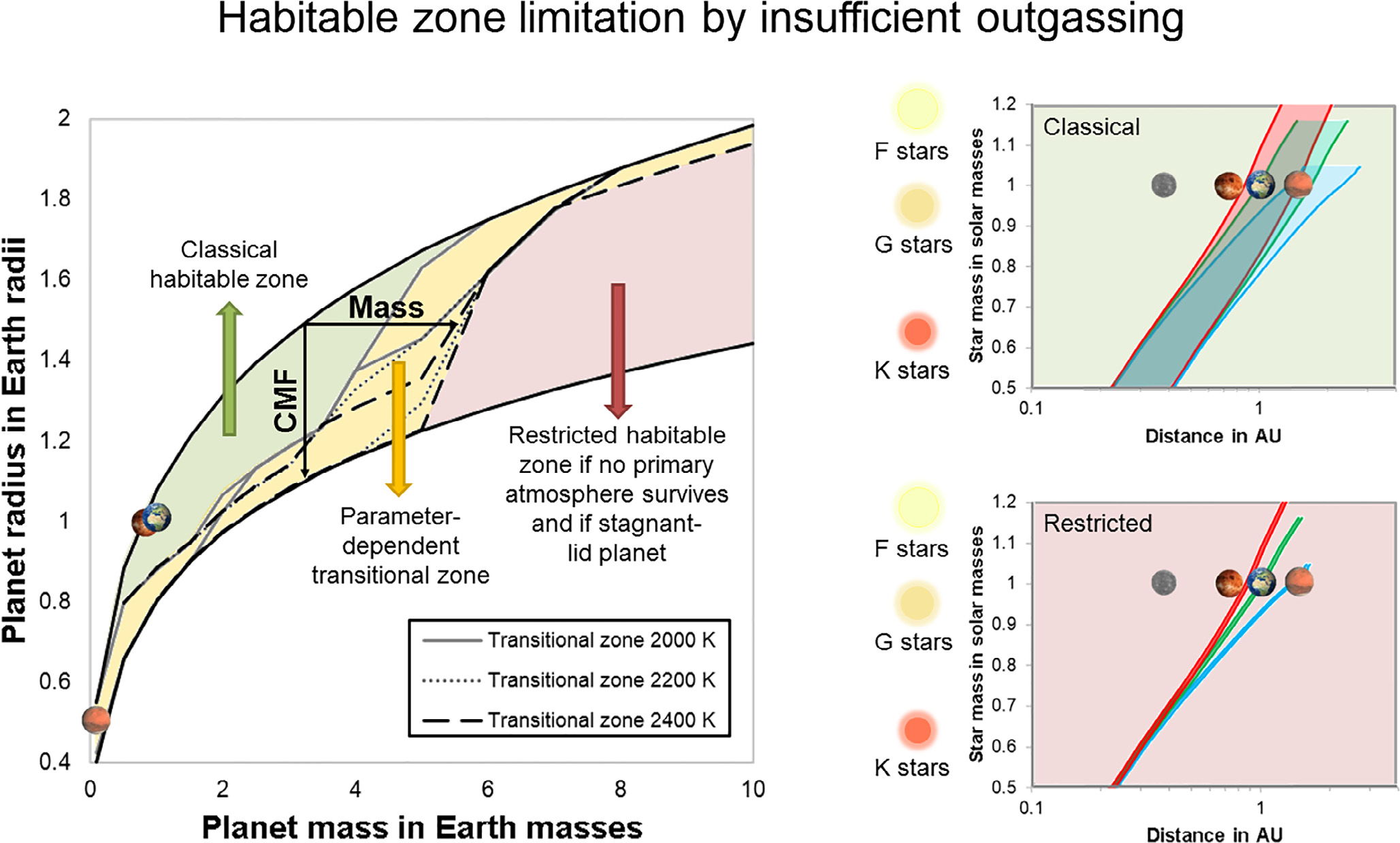 |
Volcanism and outgassing of stagnant-lid planets: Implications for the
habitable zone
Rocky exoplanets are typically classified as potentially habitable planets, if liquid water exists at the surface.
The latter depends on several factors like the abundance of water but also on the amount of available
solar energy and greenhouse gases in the atmosphere for a sufficiently long time for life to evolve.
The range of distances to the star, where surface water might exist, is called the habitable zone. Here we
study the effect of the planet interior of stagnant-lid planets on the formation of a secondary atmosphere
through outgassing that would be needed to preserve surface water.
We find that volcanic activity and associated outgassing in one-plate planets is strongly reduced after
the magma ocean outgassing phase for Earth-like mantle compositions, if their mass and/or core-mass
fraction exceeds a critical value. As a consequence, the effective outer boundary of the habitable zone
is then closer to the host star than suggested by the classical habitable zone definition, setting an important
restriction to the possible surface habitability of massive rocky exoplanets, assuming that they did
not keep a substantial amount of their primary atmosphere and that they are not in the plate tectonics
regime.
|
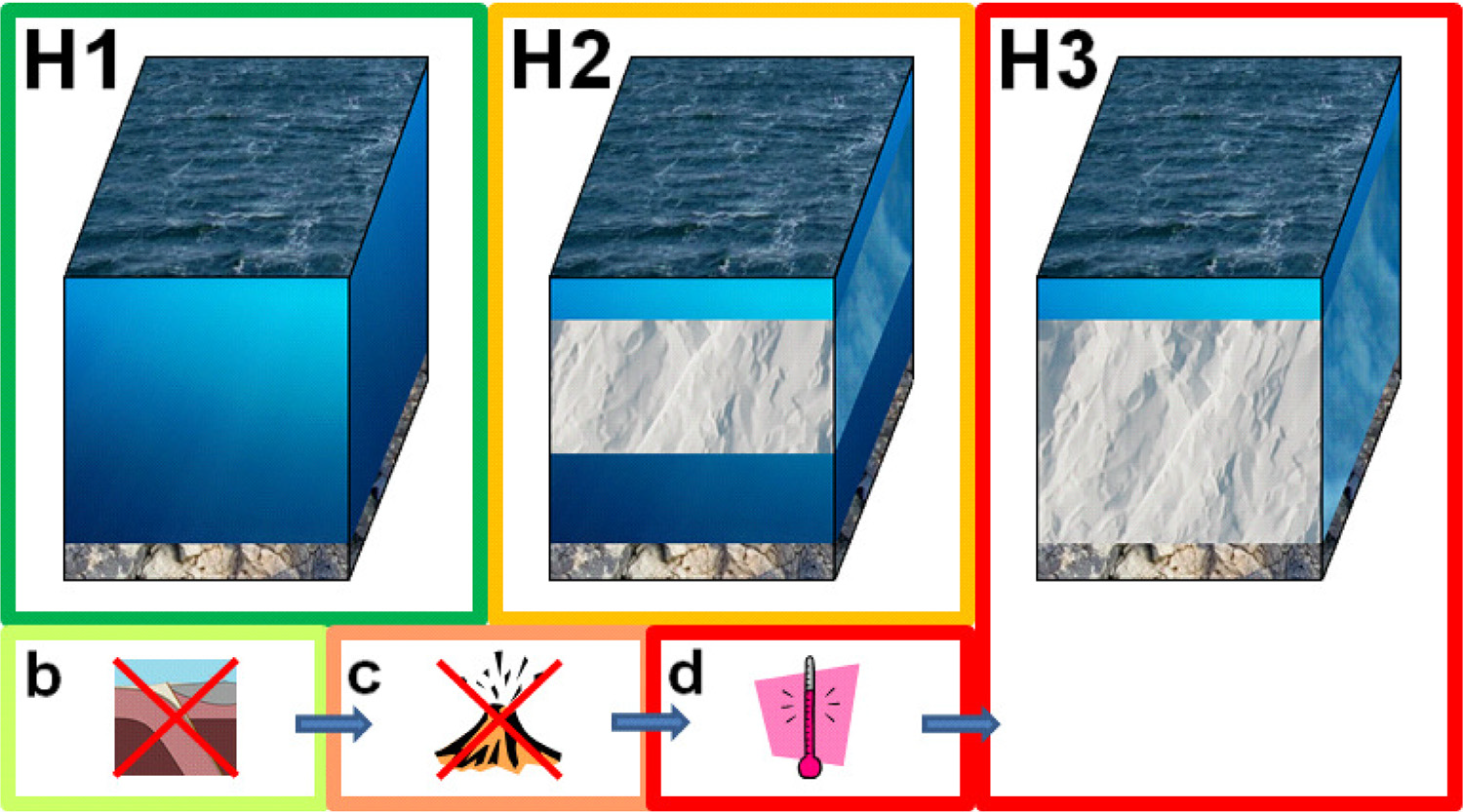 |
Water-rich planets: how habitable is a water layer deeper than on Earth?
Water is necessary for the origin and survival of life as we know it.
We study possible constraints for the habitability of deep water layers and introduce a new habitability classification relevant for water-rich planets (from Mars-size to super-Earth-size planets). A new ocean model has been developed that is coupled to a thermal evolution model of the mantle and core. We find that heat flowing out of the silicate mantle can melt an ice layer from below (in some cases episodically), depending mainly on the thickness of the ocean-ice shell, the mass of the planet, the sur- face temperature and the interior parameters (e.g. radioactive mantle heat sources). We conclude that water-rich planets with a deep ocean, a large planet mass, a high average density or a low surface temperature are likely less habitable than planets with an Earth-like ocean.
|